At 108 Performance, our training is centered around two bedrock principles:
- All humans are pre-programmed for reciprocal movement
- All humans are living and breathing biotensegrity systems
This article is going to focus on the second principle: What in the world is biotensegrity and why in the world is it important when it comes to training baseball players?
If you’ve followed along with any of our content before, there’s a good chance you understand just how important biotensegrity is to us. Our research into the concept has completely changed how we train athletes at 108. If you’ve never heard of biotensegrity before, that’s okay. There are things coaches will never need to know when it comes to the complexities and intricacies of biotensegrity. However, there are some things all coaches need to know. Keep an open mind and stay with me over the next 1,600 words. Your beliefs on how you train baseball athletes might completely change – just the way ours did.
Before we dive into what “biotensegrity” means, let’s strip off the first three letters and start with the concept of “tensegrity.”
Tensegrity, coined by Buckminster Fuller in the 1960s, is short for “tensional integrity.” If you couldn’t have guessed, two elements must be met for something to have tensegrity: Tension and Integrity. These structures have a couple of key design patterns:
- Rods and cables are loaded in pure compression/tension. The structure only fails if the cables yield or the rods buckle.
- The constant compression creates a “preload” or tensional “prestress.” This allows for structural integrity.
- When tension increases, the structure becomes stiffer. This is because the constant compression gives the system a bandwidth to disperse stress without losing structural integrity.
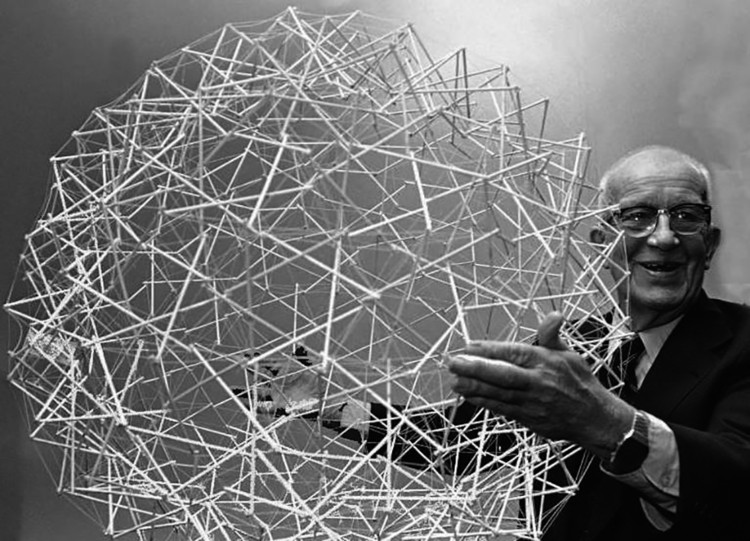
If we want to build something that lasts the test of time, we must go to the laws of evolution. Serge Gracovetsky, world renowned researcher and author of The Spinal Engine, discovered the fittest of a species survives the longest because it expends the least amount of energy to satisfy its needs. They make most efficient and economical use of their energy and resources. Tensegrity models are no different. They withstand the most amount of stress using the least amount of material. There are no moments where rods become sheared or one section takes on more than what it can handle. Everything works together to evenly disperses stress throughout the entire system and maintain integrity when exposed to load, pressure, or vibration. To put it very simply: They are efficient.
A great metaphor for tensegrity models is to think about what happens when you compress a deflated basketball. If you apply pressure to one part of it, the entire basketball stiffens and becomes more stable. This is exactly when happens in a tensegrity structure. When you press on one part of the structure, the entire structure becomes stiffer and absorbs the load being placed on the system. This is architectural efficiency.
“All structures balance tension with compression – the yin and yang of biomechanics. Tensegrity engineering uniquely positions isolated compression members within a balancing sea of tension.” – Thomas Myers, fascia researcher and author of Anatomy Trains
Now let’s add the prefix “bio” back on to tensegrity.
Biotensegrity is a term coined by Dr. Stephen Levin. Through his observations, Levin came to the understanding that our model for human movement was inadequate. Humans do not execute tasks as a system of fulcrums and levers that operate in a single plane of motion. They are biological systems that move omnidirectionally and are capable of sustaining loads that would snap most levers in half. He concluded tensegrity structures were the only thing possible that could explain how biological systems move, accept, and produce force through various vectors. He added the prefix “bio” and came up with the word “biotensegrity.”
A really easy way to think of this is to view our bones as the rods in a tensegrity system. While the bones give us structure and rigidity, they are not capable of producing movement on their own. As a result, we have to go to the “cables” of our body to understand the role tensegrity plays in biological systems. These cables are better known as fascia. If you remember anything from this article, remember fascia. It will absolutely challenge your current thoughts on how to train baseball athletes.
What is Fascia?
A great analogy for fascia is to think of it as our human “spiderman suit.” It’s as thin as thread, strong as steel, and composed largely (90 percent) of collagen and water. Fascia wraps around everything in our body and inserts into everything from muscles, bones, organs, and ligaments. This body wide sheet of connective tissue creates a continuous web of tension that turns our body into a giant tensegrity system. If we want to understand how our body is able to function as one, interconnected system, the conversation has to start with fascia. It’s the only thing that actually connects everything inside of our body.
Fascia has three key components, as outlined by Bill Parisi in his book Fascial Training:
- Viscosity
- As mentioned above, fascia is almost entirely composed of collagen and water. The more hydrated fascia is, the easier it can slide against itself. If you’ve ever had a knot in your back before, you know what it feels like when it can’t.
- Elasticity
- The fascial system gives us the ability to create elastic energy. This elasticity is more efficient than muscular power, allows for greater motor recruitment, and is responsible for up to 30 percent of our explosive power. There’s a reason why research has shown kangaroos actually expend less energy hopping than walking. Their legs have been fine tuned into explosive springs that leverage the elastic “recoil” effect created by fascia.
- Plasticity
- Fascia is a form of connective tissue. According to Davis’ Law, all connective tissue remodels and reshapes in accordance with the stresses under which it is placed. Thus, fascia can get stronger just the way your muscles get stronger after you lift weights.
If you want to create force fast and expend the least amount of energy possible, you don’t want to rely primarily on the muscular system. It’s inefficient. You need to use the elastic properties of the fascial system.
“The best athletes don’t test the highest in terms of strength. They are, however, the best mechanical composites.” – Dr. Stuart McGill, world renowned researcher and author on back pain rehabilitation, injury prevention
With up to 10 times the amount of proprioceptors and six times more sensory nerve endings, fascia is the ultimate communication system. The problem is we don’t communicate with it nearly enough. In Parisi’s opinion, most training programs spend too much time in the sagittal plane and focus on moving heavy external loads. This is the opposite of how you train the fascial system.
Instead, fascia needs to be trained doing two things:
- Submaximal loading
- Omnidrectional
If you’ve ever been around an athlete with “farm strength,” this is where it comes from. The chores they do are submaximal loads done through multiple planes of motion. Our training with baseball athletes should take a very similar approach. After all, the baseball ball weighs only five ounces and an average bat weighs two pounds. Having success on the diamond depends on our ability to move submaximal loads omnidirectionally.
Back squatting might help our muscular system get stronger, but it doesn’t impact the fascial system in the same way. This is something Parisi cautioned against. Most of the soft tissue injuries he sees were created because the muscular system was prioritized over the fascial system. If there is an imbalance between muscles and fascia, something will break until that balance is restored. This often happens after it’s too late.
In order to avoid these imbalances, we need to train the fascial system to withstand the demands of the sport. This starts, in Parisi’s opinion, by laying down lines of stress outside of the center of mass.
“We are always facilitating muscles when we contract,” said Parisi. “It’s about co-contracting muscles along the fascial lines outside of the center of mass. You’re trying to create a different stress on the body that it is not accustomed to, but it will see in sport.”
These lines are better known as fascial slings. At 108, we view these slings under two big buckets:
- The Engine
- The Brakes
Think of the brakes and the engine as the yin and the yang of the fascial network. While one is pushing, the other is pulling. When one is rotating, the other is resisting rotation. This system of checks and balances helps facilitate the co-contractions of key muscles along the fascial lines. This creates stability for your joints so the distal segments (e.g. arms, legs) can mobilize and execute fine motor tasks like throwing a baseball, for example.
The problem becomes when these systems can no longer keep each other in check. Strong engines must be paired with a strong set of brakes. If they cannot, something else is going to have to take on more than it can handle. This throws the entire tensegrity structure out of balance and puts your health and performance at risk. If you’ve ever been around an athlete with some serious juice who had lower back pain, difficulties staying on breaking balls, and constantly yanked homers foul, you know exactly what this looks like.
Let’s recap:
- Biotensegrity comes from the term “tensegrity” which refers to a unique system of cables and rods loaded in a web of compression. They use the least amount of material to withstand the most amount of stress.
- When tensegrity structures are exposed to pressure, load, or vibration, they become stiffer and disperse the stress throughout the entire system.
- Humans are not mechanical systems that operate under fulcrums and levers. They are biological systems that are capable of moving omnidirectionally and producing force through various vectors.
- Fascia has three components: Viscosity, Elasticity, and Plasticity.
- The “elastic recoil” effect created by the fascia system allows for greater motor recruitment than muscles and accounts for up to 30 percent of our explosive power.
- Fascia is trained doing two things: Moving submaximal loads and moving them omnidirectionally.
- Good fascial training lays down lines of stress outside of our center of mass which work to create co-contractions of key muscles during specific movements.
- The “engine” and “brakes” of our fascial system work to produce and accept force during dynamic movements while keeping our tensegrity structure intact.
Ready to hear how we train athletes using the principles of biotensegtity at 108?
That’ll have to wait for next time.